Immunological, oxidative, and structural factors and their responses to regulatory t lymphocyte therapy in amyotrophic lateral sclerosis
Abstract
Aim: Amyotrophic lateral sclerosis (ALS) is a systemic disease in which multiple dysfunctional pathways converge, culminating as this devastating disease. Immunological, oxidative, and structural analytes in cross-sectional and longitudinal patient sera samples were investigated and evaluated for their responses following autologous regulatory T lymphocyte (Treg)/IL-2 therapy.
Methods: This retrospective cohort study was conducted at Houston Methodist Hospital and Massachusetts General Hospital in adults with sporadic ALS. Cross-sectional and longitudinal sera levels for each of the immunological (CCL2, IL-18), oxidative (4-HNE, MDA), and structural analytes (Nf-L, pNf-H) were assayed by ELISAs, and correlated with disease progression and clinical outcomes.
Results: CCL2 and IL-18 levels were elevated in patients, especially rapidly progressing patients. 4-HNE was elevated in a subset of patients, whereas MDA was elevated in cross-sectional and longitudinally studied subjects. Nf-L was elevated in rapidly progressing patients, whereas pNf-H was decreased in these same patients. In the eleven patients assayed longitudinally, only three patients had increased levels of Nf-L or pNf-H; no patient had increased levels of both neurofilaments. Treg/IL-2 therapy suppressed levels of CCL2, IL-18, and 4-HNE.
Conclusions: In these cohorts of patients with sporadic ALS, CCL2, IL-18, and 4-HNE accurately reflected disease progression on and off therapy; MDA was elevated but did not respond to therapy. The cross-sectional and longitudinal data were complementary. Nf-L and pNf-H did not reliably and consistently reflect disease progression. Immunological and oxidative pathological factors accurately reflected therapeutic responses in these pathways and are candidates to target clinical trial endpoints.
Keywords
INTRODUCTION
Amyotrophic lateral sclerosis (ALS) is characterized by heterogeneous clinical presentations and rates of progression among affected individuals[1,2]. Inflammation in the central nervous system (CNS) of patients with ALS is a prominent pathological observation and contributes to disease progression[2]. The discovery of risk genes involved in the pathologic signaling suggests that inflammation is critical for the onset and progression of ALS; the linkage of these risk genes to ALS provides evidence that immune dysregulation contributes to ALS pathogenesis[1]. The structural integrity of the blood-brain barrier is also impaired in the presence of ALS pathology, thereby permitting substantial crosstalk between the CNS and peripheral immune systems[3,4]. Recent studies suggest that ALS is a multifactorial, multisystem disease where inflammation, in both the periphery and CNS, plays an important role in the development and progression of disease[1,5-9]. Thus, circulating immune cells might reflect and contribute to ALS pathogenesis.
Pathological hallmarks of ALS include alterations in multiple biological pathways including inflammation, oxidative stress (OS), and neuronal structural integrity; abnormal calcium entry at axon terminals induces mitochondrial dysfunction, which in turn induces OS and inflammation that damage neuronal integrity[10]. Mitochondria, due to their main role in oxidative ATP production, are important sites of intracellular reactive oxygen species (ROS) generation. Increased ROS and overwhelmed antioxidant defenses induce mitochondrial DNA damage, leading to mitochondrial dysfunction and enhanced OS; OS is an important pathological feature in ALS and is generated by the disparity between the production of ROS and their elimination by protective mechanisms, which leads to chronic inflammation[11]. Evidence of oxidative damage in patients with ALS includes increases in malondialdehyde (MDA)-modified proteins and 4-hydroxy-2-nonenal (4-HNE) protein conjugates, products of lipid peroxidation[11].
Earlier reports demonstrated that peripheral blood components of patients with ALS cluster into a distinct profile based upon cytokine levels such as interleukin (IL)-6, interleukin(IL)-1, and tumor necrosis factor – alpha (TNF-α), as well as the cellular constituents including neutrophils, CD4 T lymphocytes, natural killer-like T lymphocytes, and monocytes[12-14]. This profile correlates with disease progression and implicates a peripheral systemic pro-inflammatory response. Furthermore, patients with ALS have chronic and persistent low-grade systemic pro-inflammatory responses with the production of specific proteins involved in inflammation that correlate with their disease burdens; a heightened systemic inflammatory state is associated with a worse prognosis in ALS[15].
Because of their selective vulnerability, neurons exhibit functional decline and cell death due to OS in neurodegenerative diseases such as ALS[16]. Inflammation is also associated with increased neurofilament levels. Regression models supported the hypothesis that IL-6 and neurofilaments were both negatively associated with brain degeneration and consistent with the idea that neurofilaments are indicative of neuronal damage[17,18]. OS contributes to axonal damage through impairment of mitochondrial function, which leads to energetic failure, protein and lipid oxidation, and microtubule/neurofilament degradation. Thus, lipid peroxides and OS promote inflammation, and pro-inflammatory responses promote OS, a vicious cycle leading to the eventual degradation of neuronal structure[10].
These diverse pathways are variably compromised in ALS, making it difficult to determine whether and when potential therapies would be most efficacious. This emphasizes the need to enhance clinical trial design so that each therapy targets the appropriate pathway at the earliest disease stage. However, these biological pathways should not be interpreted in the context of individually isolated pathways but viewed as a convergence of multiple dysfunctional pathways that ultimately lead to ALS. Thus, immunological, oxidative, and structural factors need to be assessed simultaneously to monitor disease progression and to properly target clinical trial endpoints.
METHODS
Subjects
Study design and subject selection have been previously described[19,20]. For the cross-sectional cohort of sporadic ALS patients (n = 30), demographic characteristics included an average age of 58.8 years [standard deviation (SD) = 1.57]. Of this group, 63.3% were male, while the remaining 36.7% were female. Out of the total, 86.7% were identified as white, while 6.7% were categorized as Hispanic, with 3.3% being classified as black, and an additional 3.3% falling into the Asian category. Among the healthy control (HC) participants (n = 10), age demographics were comparable, with an average age of 57.6 years (standard deviation [SD] = 2.15). Within this group, 60% were male and 40% were female. Regarding racial distribution, 90.0% were identified as white, with no individuals identifying as Hispanic or black. Additionally, 10% were classified as Asian.
Clinical trials
Clinical trial designs and methods have been previously reported[21,22]. Both clinical trials obtained approval from the FDA and Houston Methodist Hospital’s (HMH) IRB prior to study initiation; written informed consent was obtained from all participants before enrollment. These two trials were registered on clinicaltrials.gov (NCT03241784 and NCT04055623). Briefly, for the phase I trial, 3 subjects with ALS were chosen entrenched their differing sites of disease - arm, bulbar, and leg - onset and progression rates[21]. Leukapheresis was performed one month before the first Treg infusion. Tregs were isolated and expanded according to a previously described protocol[23]. Subjects underwent a total of eight infusions of
ELISAs
Immunological [monocyte chemoattractant protein-1 (CCL2) and interleukin(IL)-18], OS [4-hydroxy-2-nonenal (4-HNE) and malondialdehyde (MDA)], and structural [neurofilament light chain (Nf-L) and phosphorylated neurofilament heavy chain (pNf-H)] were assayed in sera of patients and HC by ELISA according to manufacturer’s instructions.
Statistics
ANOVA was employed to conduct comparisons involving more than two groups, while comparisons between two groups were carried out using the Student's t-test. The ANOVA analysis was displayed with the degrees of freedom, F value, and corresponding P value. As for the Student’s t-test, only the p value was showcased. Spearman Rank Order correlation analysis was performed utilizing SigmaStat software, and the results were exhibited in terms of rho (r) and associated P values.
RESULTS
Immunological analytes
Using a blinded and impartial assay, it was observed that patients with sporadic ALS (n = 30) exhibited an elevated level of soluble CCL2 compared to the age-matched healthy controls (n = 10) (P < 0.001), as depicted in Figure 1A. Upon categorizing the patients into those with rapid and gradual disease progression, it was noted that CCL2 levels were increased in individuals experiencing rapid progression (n = 14) in comparison to those with a slower progression (n = 16) (P = 0.004), as well as compared to the healthy controls (P < 0.001) [F(2, 37) = 16.43, P < 0.001]. Additionally, CCL2 levels were higher in the slowly progressing patients when contrasted with the healthy controls (P = 0.003), as illustrated in Figure 1B.
Figure 1. Inflammatory analytes. (A) CCL2 was elevated in sera of patients with ALS compared with sera from age-matched HC); (B) CCL2 was elevated in rapidly progressing patients compared with slowly progressing patients; CCL2 was elevated in slowly progressing patients compared with HC; (C) IL-18 was elevated in patients compared with HC; (D) IL-18 was elevated in rapidly progressing patients compared with slowly progressing patients; IL-18 was elevated in slowly progressing patients compared with HC; (E - G) CCL2 and IL-18 correlated with disease progression but did not correlate with each other. *P < 0.05, ** P < 0.01, *** P < 0.001, and n.s.: not significant.
IL-18 was elevated in patients compared with HC (P < 0.001) [Figure 1C]; IL-18 was elevated in rapidly progressing patients compared with slowly progressing patients (P = 0.004) or HC (P < 0.001) [F(2, 38) = 12.19, P < 0.001] [Figure 1D]. CCL2 and IL-18 correlated with disease progression
OS analytes
A marker of OS, 4-HNE, was elevated in patients compared with HC (P = 0.006) [Figure 2A][10]. 4-HNE was elevated in rapidly progressing patients compared with slowly progressing patients (P = 0.003) or HC (P < 0.001) [F(2, 37)=12.05, P <0.001]; 4-HNE was not elevated in slowly progressing patients compared with HC [Figure 2B][10].
Figure 2. Oxidative stress analytes. (A) 4-HNE was elevated in patients compared with HC; (B) 4-HNE was elevated in rapidly progressing patients compared with slowly progressing patients; 4-HNE was not elevated in slowly progressing patients compared with HC; (C) MDA was elevated in patients compared with HC; (D) MDA levels were elevated and comparable in both rapidly and slowly progressing patients; levels in both were elevated compared with HC; (E - G) 4-HNE positively correlated with disease progression, but MDA levels did not correlate with progression. 4-HNE and MDA did not correlate with each other. ** P < 0.01, *** P < 0.001, and n.s.: not significant.
A second marker of OS, MDA, was elevated in patients compared with HC (P < 0.001) [Figure 2C]. MDA levels were elevated and comparable in both rapidly and slowly progressing patients and levels in both were elevated compared with HC (P < 0.001) [F(2, 37) = 38.74, P < 0.001] [Figure 2D]. 4-HNE positively correlated with disease progression (r = 0.605, P < 0.001), but MDA levels did not correlate with progression (r = 0.076, P = 0.688); 4-HNE and MDA did not correlate with each other (r = -0.047, P = 0.805) [Figure 2E-G].
Structural analytes
Nf-L was not elevated in sera of cross-sectionally studied patients [Figure 3A] but was elevated in rapidly progressing patients compared with slowly progressing patients (P = 0.038) [F(2, 37) = 3.28, P = 0.049]; Nf-L was not elevated in rapidly and slowly progressing patients compared with HC [Figure 3B]. pNf-H was not elevated in patients compared with HC but was decreased in rapidly progressing patients compared with HC (P < 0.001) [F(2, 37) = 2.58, P = 0.089]; there was no difference in pNf-H levels between slowly progressing patients and HC [Figure 3C and D]. There was a correlation between Nf-L and disease progression (r = 0.395, P = 0.031), but there was a negative correlation between pNf-H and disease progression (r = -0.512, P = 0.004); the neurofilaments did not correlate with each other (r = -0.233, P = 0.216) [Figure 3E-G].
Figure 3. Structural analytes. (A and B) Nf-L was not elevated in the sera of studied cross-sectional patients, but was elevated in rapidly progressing patients compared with slowly progressing patients; Nf-L was not elevated in both rapidly and slowly progressing patients compared with HC; (C and D) pNf-H was not elevated in cross-sectionally studied patients compared with HC, but was decreased in rapidly progressing patients compared with HC; there was no difference in pNf-H levels between slowly progressing patients and HC; (E - G) There was a weak correlation between Nf-L and disease progression, but there was a negative correlation between pNf-H and disease progression; Nf-L and pNf-H did not correlate with each other. * P < 0.05, *** P < 0.001, and n.s.: not significant.
Analytes and their responses in a Treg/IL-2 phase I trial
Clinical disease progression and disease burden were assessed using the quantitative Appel ALS (AALS) scoring system; this system permits initial trials for therapies in ALS by comparison of relatively small groups of treated patients to this historical control group[25]. Treg/IL-2 combination therapy suppressed CCL2 and IL-18 levels with a concomitant increase in both when not on Treg therapy but still receiving IL-2 in two of three phase I subjects [Figure 4A and B][21]. During the six-month Treg “washout” period, when subjects were receiving only IL-2, CCL2 and IL-18 levels rose towards the baseline levels with a coinciding deterioration of the AALS clinical score. With a second round of infusions, administered every four weeks for a total of four infusions, the therapy suppressed CCL2 and IL-18 levels and the subjects’ clinical statuses stabilized. At the end of the study when Treg infusions ceased and only IL-2 was continued, CCL2 and IL-18 levels gradually increased again. The stabilization and deterioration of the subjects’ clinical statuses mirrored the decline and rise of serum CCL2 and IL-18 levels. The third subject had increased levels of CCL2 that was refractory to Treg/IL-2 therapy; IL-18 was not elevated in subject three throughout the study [Figure 4C]. However, similar to the other two subjects, subject three’s progression stabilized when receiving Treg/IL-2 therapy but progressed again when Treg therapy was stopped and only IL-2 was administered. Treg infusions suppressed serum 4-HNE levels, but they rose during the “washout” period in two of the three phase I subjects [Figure 5A and B]. Subject three did not have elevated 4-HNE levels throughout the study [Figure 5C]. MDA was longitudinally elevated in the sera of all three subjects throughout the study and the levels were refractory to Treg/IL-2 therapy. Nf-L and pNf-H levels were not elevated in two of the three longitudinally studied phase I subjects [Figure 6A and B]; although Nf-L was not elevated in subject 3, pNf-H was elevated [Figure 6C].
Figure 4. (A and B) Treg/IL-2 combination therapy suppressed CCL2 and IL-18 levels with a concomitant increase in both when not on Treg therapy but still receiving IL-2 in two of three phase I subjects. During the six-month Treg “washout” period, when subjects were receiving only IL-2, CCL2 and IL-18 levels rose towards the baseline level with a coinciding deterioration of the AALS. With a second round of infusions, administered every four weeks for a total of 4 infusions, the therapy suppressed CCL2 and IL-18 levels and the subjects’ clinical statuses stabilized. At the end of the study when Treg infusions ceased and only IL-2 was continued, CCL2 and IL-18 levels gradually increased again. The stabilization and deterioration of the subjects’ clinical statuses mirrored the decline and rise of serum CCL2 and IL-18 levels; (C) The third subject had increased levels of CCL2 that was refractory to Treg/IL-2 therapy; IL-18 was not elevated in subject 3 throughout the study. However, similarly to the other two subjects, subject three’s progression stabilized when receiving Treg/IL-2 therapy but progressed again when Treg therapy was stopped and only IL-2 was administered. Arrows indicate Treg infusion dates. The red dotted lines demarcate Treg + IL-2 therapy or IL-2 only intervals. Red line = mean value of each analyte in HC. Black lines ± one standard deviation of each analyte level in HC. The means and standard deviations vary due to the differences in ELISA plates used. AALS: Appel ALS clinical scoring system.
Figure 5. (A and B) Treg infusions suppressed serum 4-HNE levels, but they then rose during the “washout” period when not on Treg therapy in two of the three phase I subjects;. (C) Subject three did not have elevated 4-HNE levels throughout the study. MDA was longitudinally elevated in the sera of all three subjects throughout the study and the levels were not altered by Treg therapy. Arrows indicate Treg infusion dates. The red dotted lines demarcate Treg + IL-2 therapy or IL-2 only intervals. Red line = mean value of each analyte in HC. Black lines ± one standard deviation of each analyte level in HC. The means and standard deviations vary due to the differences in ELISA plates used. AALS: Appel ALS clinical scoring system.
Figure 6. (A - C) Nf-L and pNf-H levels were not elevated in two of the three phase I trial subjects, but pNf-H was elevated in subject three; Nf-L was not elevated and did not respond to Treg/IL-2 therapy in subject three. Arrows indicate Treg infusion dates. The red dotted lines demarcate Treg + IL-2 therapy or IL-2 only intervals. Red line = mean value of each analyte in HC. Black lines ± one standard deviation of each analyte level in HC. The means and standard deviations vary due to the differences in ELISA plates used. AALS = Appel ALS clinical scoring system.
Analytes and their expressions during a Treg/IL-2 phase IIA trial
Treg/IL-2 combination therapy suppressed CCL2 levels in five of the eight phase IIA subjects [Figure 7A and B]; the analytes were separated and grouped based on both the basal expression levels and whether the analyte levels increased or had no change and whether the expression levels decreased or had no change[22]. Subjects 103 and 201 were rapidly progressing, whereas 202 was progressing at an intermediate rate, and in all three subjects, their CCL2 expression levels were elevated above that observed with HC; in subjects 114, 115, 203, 205, and 206, CCL2 expression levels were low and their levels remained within HC levels throughout the study. Treg/IL-2 combination therapy suppressed IL-18 levels in six of the eight phase IIA subjects. IL-18 levels in two phase IIA subjects, 103 and 202, were elevated throughout the study; the remaining phase IIA subjects’ IL-18 levels were low and only rose following cessation of Treg therapy [Figure 7C and D]. 4-HNE levels in phase IIA subjects 103 and 201 were elevated; 4-HNE levels in the remaining six phase IIA subjects were low and only rose following cessation of therapy [Figure 7E and F]. MDA levels were refractory to Treg/IL-2 therapy in seven of the eight phase IIA subjects; only subject 115’s MDA levels were low throughout the study [Figure 7G and H]. Interestingly, only one phase IIA subject, 201, had increased Nf-L levels and those levels increased during the study; the remaining phase IIA subjects’ Nf-L levels remained within HC levels throughout the study [Figure 7I and J]. As with Nf-L levels, only one phase IIA subject had increased levels of pNf-H. Curiously, the same phase IIA subject did not have elevated levels of both Nf-L and pNf-H in their sera, but they were the two subjects who did not experience stabilization of disease progression while receiving Treg/IL-2 therapy[24]. The remaining seven phase IIA subjects’ pNf-H levels remained within HC levels throughout the study [Figure 7K and L]. Thus, only one of eight subjects in the phase IIA trial had an elevated level of Nf-L, whereas a different sole subject had an elevated level of pNf-H; none of the eleven subjects studied longitudinally had elevated levels of both Nf-L and pNf-H. Only three samples, out of the twenty-two samples studied longitudinally, had elevated levels of either Nf-L or pNf-H (14%).
Figure 7. (A and B) CCL2 levels were suppressed in five of the eight phase IIA subjects. CCL2 levels in subjects 103, 201, and 202 were elevated above that observed with HC; in the remaining subjects, CCL2 expression levels were low and their levels remained within HC levels; (C and D) Treg/IL-2 combination therapy suppressed IL-18 levels in six of the eight phase IIA subjects. IL-18 levels in two phase IIA subjects, 103 and 202, were elevated throughout the study; the remaining phase IIA subjects’ IL-18 levels were low and only rose following cessation of Treg therapy; (E and F) 4-HNE levels in phase IIA subjects 103 and 201 were elevated; 4-HNE levels in the remaining six phase IIA subjects were low and only rose following cessation of therapy; (G and H) MDA levels were refractory to Treg/IL-2 therapy in seven of the eight phase IIA subjects; only subject 115 had low MDA levels throughout the study; (I and J) Only phase IIA subject, 201, had increased Nf-L levels and those levels increased throughout the study; the remaining phase IIA subjects Nf-L levels remained within HC levels; (K and L) Only one phase IIA subject had increased levels of pNf-H. The remaining seven phase IIA subjects remained within HC levels throughout the study. Red line = mean value of each analyte in HC. Black lines ± one standard deviation of each analyte level in HC. The phase IIA trial consisted of a 24-week randomized, double-blind, placebo-controlled trial (RCT) followed by a 24-week ascending dose open-label extension (OLE)[24]. Blue arrows indicate when subjects received Treg/IL-2 therapy during the RCT and the red arrows indicate when subjects received Treg/IL-2 therapy during the OLE. Four subjects, 103, 115, 202, and 204, were randomized to the placebo group during the RCT but received Treg/IL-2 infusions during the OLE. Three subjects, 114, 201, and 203, were randomized to the active group and received Treg/IL-2 therapy during the RCT and later received Treg/IL-2 infusions during the OLE. Two subjects, 205 and 206, were directly enrolled in the OLE and received Treg/IL-2 therapy[24].
DISCUSSION
In the progression of ALS, there is a simultaneous and increasing cascade of pathological responses involving inflammation, oxidative stress, and neuronal structural fragmentation. These culminate in an intensified disease burden[17,18,26]. The motoneuron projects outside the blood-brain barrier to the muscle and the axon terminal may be one of the early sites of pathology, initiating a “dying back” phenomenon[10,27]. Increased calcium entry at axon terminals induces mitochondrial dysfunction, which in turn induces OS and inflammation that damage neuronal integrity and provoke an acute phase response (APR) with increased expression of acute phase proteins (APP)[28,29]. The APR accompanies chronic as well as acute inflammatory states. Pro-inflammatory cytokines, including IL-6, IL-1β, and TNF-α, induce the expression of APPs, which are synthesized by and secreted from liver hepatocytes[28]. Thus, OS promotes inflammation, and pro-inflammatory responses promote APP expression and further OS, culminating in a vicious cycle of injury leading to the eventual degradation of neuronal axonal structure.
Thonhoff et al. (2022) state that in multiple diseases, Tregs lose their suppressive function and their neuroprotective capabilities and that when Tregs are expanded ex vivo in the presence of interleukin (IL)-2 and rapamycin, the dysfunctional Tregs regain their suppressive function[22]. Thus, in theory, Treg/IL-2 treatments should suppress the escalating cascade of pro-inflammatory/oxidative stress responses that develop in patients with ALS; Tregs have the potential to suppress inflammation in both the periphery and CNS immune compartments. With this physiological response in mind due to Treg/IL-2 therapy, again, in theory, the structural destruction of axons should be reduced with the concomitant reduction in serum levels of Nf-L and pNf-H.
This report demonstrates that serum soluble inflammatory analytes, CCL2 and IL-18, were elevated not only in cross-sectional patient samples but also in longitudinal patient samples; the cross-sectional and longitudinal data are complementary. Previous studies were the catalyst for why CCL2 was investigated in the current study and have established the presence of activated monocytes/macrophages in the blood of patients with ALS[30]. Henkel et al. (2004) demonstrated that mRNA for the chemokine MCP-1 (CCL2) was increased in tissues obtained from patients with ALS, and that the CCL2 protein was expressed in glia from these patients. Henkel et al. (2006) also demonstrated that the CCL2 mRNA and immunoreactivity were upregulated in neuronal and glial cells of an animal model of ALS[30].
CCL2 is a potent chemoattractant and activating peptide that is secreted by monocytes/macrophages following injury, contributing to an inflammatory response; excessive CCL2 can exacerbate injury leading to further inflammation. CCL2 attracts CCR2-expressing myeloid dendritic cells, monocytes/macrophages, and activated T lymphocytes. In parallel with CCL2, IL-18 is also a pro-inflammatory cytokine whose initial production was originally recognized in Kupffer cells, but it is now known to be constitutively expressed by many hematopoietic and non-hematopoietic cells. IL-18 can modulate both innate and adaptive immunity and its dysregulation can contribute to autoimmune or inflammatory diseases. IL-18, as with IL-1, is processed intracellularly by the NLRP3 inflammasome[31]. However, the half-life of IL-1 is short, making the detection of IL-18 expression, whose half-life is much longer, a surrogate for IL-1 expression[32]. Thus, the positive correlations of CCL2 and IL-18 with disease progression make these peptides potential markers for therapeutic responses in patients with ALS. Consistent with this study, a recent study also presented evidence that following treatment with low-dose IL-2, CCL2 levels were reduced compared to placebo[33].
In addition to CCL2 and IL-18, the current report also demonstrated that serum soluble OS peroxides, 4-HNE and MDA, were elevated not only in cross-sectional patient samples but also in longitudinal patient samples; again, the cross-sectional and longitudinal data are complementary. The primary products of lipid peroxidation are 4-HNE and MDA, both of which have been extensively studied mechanistically and in the pathology of many diseases. 4-HNE is produced by lipid peroxidation of polyunsaturated fatty acids such as arachidonic acid and is one of the most toxic products of lipid peroxidation; 4-HNE oxidized lipid products have been shown to be elevated in ALS and are elevated at early stages of the disease[34-37]. MDA is also a final product of polyunsaturated fatty acids peroxidation in cells; MDA is known to be a marker of OS[38]. Once again, arachidonic acid is in the MDA biochemical pathway, a lipid that is known to spur on inflammatory processes, and patients with ALS have a 2.5-fold higher level of arachidonic acid. Unlike 4-HNE, for which levels are elevated in rapidly progressing patients with ALS, MDA is elevated in both rapidly and slowly progressing patients with ALS, possibly suggesting that MDA is a marker for developing ALS; this thought must be studied in greater detail using a larger cohort of patients with ALS. Because the number of patients in both clinical trials was small - three in the phase I trial and eight in
MDA appears to be a mutagenic product of lipid peroxidation, whereas 4-HNE is the most toxic. On one hand, MDA has been widely used for many years as a convenient biomarker for lipid peroxidation of omega-3 and omega-6 fatty acids. On the other hand, 4-HNE is elicited either by free radicals or chemicals. The 4-HNE is produced in relatively large amounts, and is very reactive and acts as a second messenger of free radicals. 4-HNE toxicity can be explained by its rapid reactions with thiols and amino groups. 4-HNE acts as both a signaling molecule and as a cytotoxic product, causing pathophysiological consequences by the covalent modification of macromolecules; 4-HNE is one of the most physiologically active lipid peroxides. Furthermore, 4-HNE is involved in the regulation of several transcription factors such as nuclear factor erythroid 2-related factor 2, activating protein-1, NF-κB, and peroxisome-proliferator-activated receptors[39].
Ferroptosis, initiated by the failure of the glutathione-dependent antioxidant defenses resulting in unchecked lipid peroxidation, is a form of iron-dependent regulated cell death that is genetically and biochemically distinct from other forms of regulated cell death, such as apoptosis, necrosis, and necroptosis[10,38,40]. Arachidonic acid is a well-known substrate for lipid peroxidation and is indispensable for ferroptosis that is known to accelerate the metabolism of arachidonic acid and stimulate the synthesis of pro-inflammatory mediators[41,42]. Thus, arachidonic acid metabolism may be dysfunctional in ALS.
Contrary to previous reports, the current study did not confirm elevated Nf-L and pNf-H in patients with ALS. Nf-L and pNf-H were not reliably and consistently elevated, did not reflect disease progression, and did not respond to Treg therapy. The lack of elevated pNf-H is consistent with a previous report[43-45]. Paganoni et al. (2020) concluded that plasma pNF-H levels did not differ and that cerebrospinal fluid (CSF) levels have been shown to have a higher correlation with disease severity and progression in persons with ALS, plasma sampling offers a more convenient and less invasive means of measuring pNf-H levels than CSF[45]. Camu et al. (2020) also did not observe any significant differences with respect to plasma Nf-L levels following treatment with low-dose IL-2[33]. Re-analysis of the plasma samples for Nf-L levels using the Quanterix Simoa approach provided similar results; Nf-L plasma levels did not differ.
There is substantial evidence that inflammation and OS contribute decisively to disease progression and neurodegeneration in ALS[10,38]. In accordance with this concept, CCL2 and IL-18 were elevated in patients, especially rapidly progressing patients; these two immune factors positively correlated with disease progression and responded to Treg/IL-2 therapy[21,24]. Furthermore, 4-HNE levels are elevated in patients with ALS even at early stages of the disease[36]. The current study demonstrated that 4-HNE and MDA were elevated in patients with ALS, although only 4-HNE responded to Treg therapy, which supports the role of OS in ALS. Thus, the simultaneously assessed levels of immunological and oxidative factors are candidates to monitor disease progression and to properly target clinical trial endpoints response to Treg/IL-2 therapy in ALS.
DECLARATIONS
Author contributions
Access to all data in the study and took responsibility for the integrity of the data and the accuracy of the data analysis: Appel SH
share co-first authorship: Beers DR, Thonhoff JR
Conceptualization: Beers DR, Thonhoff JR, Appel SH
Data curation: Beers DR, Wen S
Formal analysis: Beers DR, Thonhoff JR
Methodology: Beers DR, Thonhoff JR, Thome AD, Faridar A, Zhao W, Appel SH
Resources: Appel SH
Writing-original draft: Beers DR
Writing-review & editing: Beers DR, Thonhoff JR, Thome AD, Faridar A, Zhao W, Appel SH
All authors read and approved the final manuscript: Beers DR, Thonhoff JR, Thome AD, Faridar A, Zhao W, Appel SH, Wen S
Availability of data and materials
Available upon request.
Financial support and sponsorship
This work was supported by the Peggy and Gary Edwards ALS Laboratory, The ALS Association, ALS Finding a Cure Foundation, and Coya Therapeutics, Inc.
Conflicts of interest
Beers declares a conflict of interest as a consultant with Implicit Bioscience. Thome declares a conflict of interest as a consultant with Coya Therapeutics, Inc. Appel declares a conflict of interest as a consultant with Implicit Bioscience and scientific advisory board chair of Coya Therapeutics, Inc. The remaining authors have no conflicts of interest.
Ethical approval and consent to participate
Not applicable.
Consent for publication
Not applicable.
Copyright
© The Author(s) 2023.
REFERENCES
1. Beers DR, Appel SH. Immune dysregulation in amyotrophic lateral sclerosis: mechanisms and emerging therapies. Lancet Neurol 2019;18:211-20.
3. Zlokovic BV. The blood-brain barrier in health and chronic neurodegenerative disorders. Neuron 2008;57:178-201.
4. Henkel JS, Beers DR, Wen S, Bowser R, Appel SH. Decreased mRNA expression of tight junction proteins in lumbar spinal cords of patients with ALS. Neurology 2009;72:1614-6.
5. Henkel JS, Beers DR, Wen S, et al. Regulatory T-lymphocytes mediate amyotrophic lateral sclerosis progression and survival. EMBO Mol Med 2013;5:64-79.
6. Lu CH, Allen K, Oei F, et al. Systemic inflammatory response and neuromuscular involvement in amyotrophic lateral sclerosis. Neurol-Neuroimmunol 2016;3:e244.
7. Beers DR, Zhao W, Wang J, et al. ALS patients’ regulatory T lymphocytes are dysfunctional, and correlate with disease progression rate and severity. JCI Insight 2017;2:e89530.
8. Zhao W, Beers DR, Hooten KG, et al. Characterization of gene expression phenotype in amyotrophic lateral sclerosis monocytes. JAMA Neurol ;74:677-85.
9. Sheean RK, McKay FC, Cretney E, et al. Association of regulatory T-cell expansion with progression of amyotrophic lateral sclerosis: a study of humans and a transgenic mouse model. JAMA Neurol 2018;75:681-9.
10. Appel SH. Oxidative stress-mediated inflammation promotes the pathogenesis of amyotrophic lateral sclerosis. Aging Neur Dis 2022;2:18.
11. Cunha-Oliveira T, Montezinho L, Mendes C, et al. Oxidative stress in amyotrophic lateral sclerosis: pathophysiology and opportunities for pharmacological intervention. Oxid Med Cell Longev 2020;2020:5021694.
12. Hu Y, Cao C, Qin XY, et al. Increased peripheral blood inflammatory cytokine levels in amyotrophic lateral sclerosis: a meta-analysis study. Sci Rep 2017;7:9094.
13. Murdock BJ, Zhou T, Kashlan SR, Little RJ, Goutman SA, Feldman EL. Correlation of peripheral immunity with rapid amyotrophic lateral sclerosis progression. JAMA Neurol 2017;74:1446-54.
14. Gustafson MP, Staff NP, Bornschlegl S, et al. Comprehensive immune profiling reveals substantial immune system alterations in a subset of patients with amyotrophic lateral sclerosis. PLoS One 2017;12:e0182002.
15. Keizman D, Rogowski O, Berliner S, et al. Low-grade systemic inflammation in patients with amyotrophic lateral sclerosis. Acta Neurol Scand 2009;119:383-9.
16. di Penta A, Moreno B, Reix S, et al. Oxidative stress and proinflammatory cytokines contribute to demyelination and axonal damage in a cerebellar culture model of neuroinflammation. PLoS One 2013;8:e54722.
17. Fischer-Hayes LR, Brotherton T, Glass JD. Axonal degeneration in the peripheral nervous system: Implications for the pathogenesis of amyotrophic lateral sclerosis. Exp Neurol 2013;246:6-13.
18. Morgan S, Orrell RW. Pathogenesis of amyotrophic lateral sclerosis. Br Med Bull ;2016:119:87-98.
19. Beers DR, Thonhoff JR, Faridar A, et al. Tregs attenuate peripheral oxidative stress and acute phase proteins in ALS. Ann Neurol 2022;92:195-200.
20. Beers DR, Zhao W, Thonhoff JR, et al. Serum programmed cell death proteins in amyotrophic lateral sclerosis. Brain Behav Immun Health 2021;12:100209.
21. Thonhoff JR, Beers DR, Zhao W, et al. Expanded autologous regulatory T-lymphocyte infusions in ALS: a phase I, first-in-human study. Neurol-Neuroimmunol 2018;5:e465.
22. Thonhoff JR, Berry JD, Macklin EA, et al. Combined regulatory T-lymphocyte and IL-2 treatment is safe, tolerable, and biologically active for 1 year in persons with amyotrophic lateral sclerosis. Neurol-Neuroimmunol 2022;9:e200019.
23. Alsuliman A, Appel SH, Beers DR, et al. A robust, good manufacturing practice-compliant, clinical-scale procedure to generate regulatory T cells from patients with amyotrophic lateral sclerosis for adoptive cell therapy. Cytotherapy 2016;18:1312-24.
24. Bluestone JA, Buckner JH, Fitch M, et al. Type 1 diabetes immunotherapy using polyclonal regulatory T cells. Sci Transl Med 2015;7:315ra189.
25. Haverkamp LJ, Appel V, Appel SH. Natural history of amyotrophic lateral sclerosis in a database population: validation of a scoring system and a model for survival prediction. Brain 1995;118:707-19.
26. Hooten KG, Beers DR, Zhao W, Appel SH. Protective and toxic neuroinflammation in amyotrophic lateral sclerosis. Neurotherapeutics 2015;12:364-75.
27. Fischer LR, Culver DG, Tennant P, et al. Amyotrophic lateral sclerosis is a distal axonopathy: evidence in mice and man. Exp Neurol 2004;185:232-40.
28. Beers DR, Zhao W, Neal DW, et al. Elevated acute phase proteins reflect peripheral inflammation and disease severity in patients with amyotrophic lateral sclerosis. Sci Rep 2020;10:15295.
29. Siklós L, Engelhardt J, Harati Y, Smith RG, Joó F, Appel SH. Ultrastructural evidence for altered calcium in motor nerve terminals in amyotropic lateral sclerosis. Ann Neurol 1996;39:203-16.
30. Henkel JS, Engelhardt JI, Siklós L, et al. Presence of dendritic cells, MCP-1, and activated microglia/macrophages in amyotrophic lateral sclerosis spinal cord tissue. Ann Neurol 2004;55:221-35.
31. Swanson KV, Deng M, Ting JP. The NLRP3 inflammasome: molecular activation and regulation to therapeutics. Nat Rev Immunol 2019;19:477-89.
32. Sutinen EM, Korolainen MA, Häyrinen J et al. Interleukin-18 alters protein expressions of neurodegenerative diseases-linked proteins in human SH-SY5Y neuron-like cells. Front Cell Neurosci 2014;8:214.
33. Camu W, Mickunas M, Veyrune JL, et al. Repeated 5-day cycles of low dose aldesleukin in amyotrophic lateral sclerosis (IMODALS): a phase 2a randomised, double-blind, placebo-controlled trial. EBioMedicine 2020;59:102844.
34. Li Y, Zhao T, Li J, et al. Oxidative stress and 4-hydroxy-2-nonenal (4-HNE): implications in the pathogenesis and treatment of aging-related diseases. J Immunol Res 2022;2022:2233906.
35. Smith RG, Henry YK, Mattson MP, Appel SH. Presence of 4-hydroxynonenal in cerebrospinal fluid of patients with sporadic amyotrophic lateral sclerosis. Ann Neurol 1998;44:696-9.
36. Simpson EP, Henry YK, Henkel JS, Smith RG, Appel SH. Increased lipid peroxidation in sera of ALS patients - a potential biomarker of disease burden. Neurol 2004;62:1758-65.
37. Devos D, Moreau C, Kyheng M, et al. A ferroptosis-based panel of prognostic biomarkers for amyotrophic lateral sclerosis. Sci Rep 2019;9:2918.
38. Park HR, Jin Yang EJ. Oxidative stress as a therapeutic target in amyotrophic lateral sclerosis: opportunities and limitations. Diagnostics 2021;11:1546.
39. Ayala A, Muñoz MF, Argüelles S. Lipid peroxidation: production, metabolism, and signaling mechanisms of malondialdehyde and 4-hydroxy-2-nonenal. Oxid Med Cell Longev 2014;2014:360438.
40. Wang T, Tomas D, Perera ND, et al. Ferroptosis mediates selective motor neuron death in amyotrophic lateral sclerosis. Cell Death Differ 2022;29:1187-98.
41. Panaroni C, Fulzele K, Soucy R et al. Arachidonic acid induces ferroptosis-mediated cell-death in multiple myeloma. Blood 2018;132:4498.
42. Kagan VE, Mao G, Qu F, et al. Oxidized arachidonic and adrenic PEs navigate cells to ferroptosis. Nat Chem Biol 2017;13:81-90.
43. Benatar M, Wuu J, Andersen PM, Lombardi V, Malaspina A. Neurofilament light: a candidate biomarker of presymptomatic amyotrophic lateral sclerosis and phenoconversion. Ann Neurol 2018;84:130-9.
44. Poesen K, Van Damme P. Diagnostic and prognostic performance of neurofilaments in ALS. Front Neurol 2019;9:1167.
Cite This Article
Export citation file: BibTeX | RIS
OAE Style
Beers DR, Thonhoff JR, Thome AD, Faridar A, Zhao W, Wen S, Appel SH. Immunological, oxidative, and structural factors and their responses to regulatory t lymphocyte therapy in amyotrophic lateral sclerosis. Ageing Neur Dis 2023;3:15. http://dx.doi.org/10.20517/and.2023.14
AMA Style
Beers DR, Thonhoff JR, Thome AD, Faridar A, Zhao W, Wen S, Appel SH. Immunological, oxidative, and structural factors and their responses to regulatory t lymphocyte therapy in amyotrophic lateral sclerosis. Ageing and Neurodegenerative Diseases. 2023; 3(3): 15. http://dx.doi.org/10.20517/and.2023.14
Chicago/Turabian Style
Beers, David R., Jason R. Thonhoff, Aaron D. Thome, Alireza Faridar, Weihua Zhao, Shixiang Wen, Stanley H. Appel. 2023. "Immunological, oxidative, and structural factors and their responses to regulatory t lymphocyte therapy in amyotrophic lateral sclerosis" Ageing and Neurodegenerative Diseases. 3, no.3: 15. http://dx.doi.org/10.20517/and.2023.14
ACS Style
Beers, DR.; Thonhoff JR.; Thome AD.; Faridar A.; Zhao W.; Wen S.; Appel SH. Immunological, oxidative, and structural factors and their responses to regulatory t lymphocyte therapy in amyotrophic lateral sclerosis. Ageing. Neur. Dis. 2023, 3, 15. http://dx.doi.org/10.20517/and.2023.14
About This Article
Copyright
Data & Comments
Data
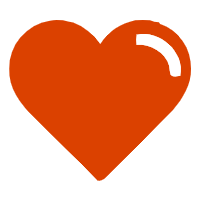

Comments
Comments must be written in English. Spam, offensive content, impersonation, and private information will not be permitted. If any comment is reported and identified as inappropriate content by OAE staff, the comment will be removed without notice. If you have any queries or need any help, please contact us at support@oaepublish.com.